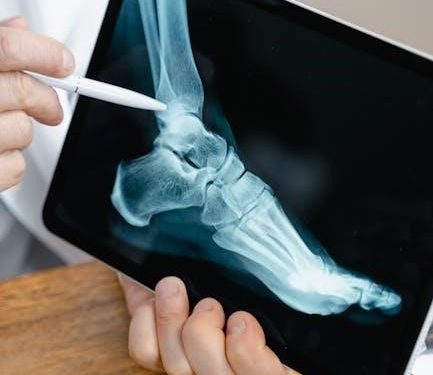
Structural analysis examines how structures respond to external loads, ensuring safety and durability. It involves understanding loads, stresses, and material behavior, essential for engineering safe buildings and bridges.
What is Structural Analysis?
Structural analysis is the process of examining how structures respond to external loads, such as weight, wind, or earthquakes. It involves calculating stresses, strains, and displacements to ensure structures are safe, stable, and durable. This analysis is fundamental in engineering, as it helps predict and mitigate potential failures.
By applying principles of physics and mathematics, structural analysis determines the behavior of materials and systems under various conditions. It integrates material properties, load distributions, and boundary conditions to provide insights into a structure’s performance. Accurate analysis ensures compliance with design standards, protecting people and infrastructure from potential hazards. Advanced tools and methodologies, such as FEM, enhance precision and efficiency in modern structural analysis.
Importance of Structural Analysis in Engineering
Structural analysis is crucial in engineering as it ensures the safety, durability, and cost-effectiveness of buildings, bridges, and other infrastructure. By evaluating how structures respond to various loads, engineers can design systems that withstand environmental and operational stresses. This process minimizes the risk of structural failures, protecting human life and property. Additionally, structural analysis optimizes material usage, reducing construction costs while maintaining performance standards. It also ensures compliance with building codes and regulations. Without structural analysis, engineers cannot guarantee the reliability of their designs, leading to potential disasters. Thus, it is a cornerstone of civil and structural engineering, enabling the creation of resilient and efficient structures.
Key Concepts in Structural Analysis
Structural analysis involves understanding fundamental principles like equilibrium, stress distribution, and deformation. These concepts ensure structures are safe and functional, forming the basis of engineering design.
Loads and Reactions
Loads are external forces acting on a structure, such as weights, impacts, or environmental forces like wind and earthquakes. Reactions are the supporting forces at the structure’s base or supports. Analyzing these forces ensures equilibrium, enabling accurate design and safety assessments. Static and dynamic loads must be considered, along with their distribution across structural elements. Proper calculation of reactions is critical for determining support requirements and ensuring structural stability. Understanding loads and reactions is fundamental to predicting how structures behave under various conditions and to ensuring their safety and functionality throughout their lifespan. This analysis forms the basis of all structural design processes.
Material Behavior and Properties
Material behavior and properties are critical in structural analysis, as they determine how materials respond to loads. Key properties include elasticity, plasticity, strength, and stiffness. Elasticity allows materials to return to their original shape after loading, while plasticity refers to permanent deformation. Strength indicates the maximum stress a material can withstand without failing, and stiffness relates to resistance to deformation. Understanding these properties helps engineers select appropriate materials for structures, ensuring durability and safety. Factors like temperature, loading rate, and environmental conditions can influence material behavior. Accurate knowledge of these properties is essential for predicting structural performance under various conditions and ensuring reliable designs. This understanding is fundamental to modern engineering practices.
Methods of Structural Analysis
Structural analysis methods include static and dynamic approaches. Static analysis evaluates equilibrium and deformation under constant loads, while dynamic analysis considers time-dependent forces and vibrations.
Static Analysis
Static analysis is a fundamental method in structural engineering used to determine the equilibrium and deformation of structures under constant loads. It involves calculating internal forces, stresses, and strains caused by external loads, ensuring the structure’s stability and safety. Static analysis assumes that loads do not change over time and focuses on the structure’s resistance to external forces. This method is widely applied in the design of beams, columns, and trusses. By understanding the distribution of forces and material behavior, engineers can ensure that structures withstand anticipated loads without failure. Static analysis forms the basis for more complex dynamic evaluations.
Dynamic Analysis
Dynamic analysis examines the behavior of structures under time-dependent loads, such as earthquakes, wind, or moving vehicles, where inertia and damping forces play a critical role. Unlike static analysis, it considers how structures respond to varying forces over time, including resonance and oscillations. This method is essential for ensuring the safety and functionality of structures subjected to dynamic excitations. Engineers use advanced techniques, including modal analysis and time-history methods, to predict structural responses. Dynamic analysis helps in designing structures that can withstand natural disasters and operational vibrations, ensuring resilience and minimizing potential damage or failure under dynamic conditions.
Structural Analysis Tools and Software
Advanced software like ETABS, STAAD.Pro, and Autodesk Robot enable precise structural simulations, ensuring optimal design and compliance with engineering standards, enhancing accuracy and efficiency in analysis.
Finite Element Method (FEM)
The Finite Element Method (FEM) is a numerical technique used to solve partial differential equations, widely applied in structural analysis to simulate stress, strain, and deformation. By discretizing a structure into smaller elements, FEM simplifies complex problems into manageable calculations. Each element’s behavior is analyzed, and results are combined to provide an overall solution. FEM excels in handling complex geometries, nonlinear material behavior, and varied load conditions. It is extensively used in engineering for designing and analyzing components like beams, plates, and 3D structures. The method ensures accurate predictions of structural performance, making it indispensable in aerospace, civil, and mechanical engineering applications.
Software Applications in Structural Analysis
Software applications play a crucial role in modern structural analysis, enabling engineers to simulate and analyze complex structures efficiently. Programs like Autodesk, SolidWorks, and ANSYS utilize finite element methods to predict stress, strain, and deformation. These tools offer 3D modeling, dynamic analysis, and material nonlinear analysis capabilities. Specialized software such as ETABS and STAAD.Pro are widely used for building and bridge design. They integrate advanced algorithms to ensure accurate simulations and compliance with design codes. Additionally, software like Abaqus and LS-DYNA handle high-performance simulations for specialized applications. These applications accelerate the design process, enhance precision, and support the creation of sustainable and resilient structures, making them indispensable in contemporary engineering practices.
Applications of Structural Analysis
Structural analysis is vital for ensuring safety and durability in building design, bridge construction, and industrial facilities, enabling engineers to create sustainable and resilient structures.
Building Design and Construction
Structural analysis is fundamental in building design and construction, ensuring structures can withstand external loads like wind, earthquakes, and gravity. It involves designing safe, durable buildings using advanced materials and techniques.
Bridge Engineering
Structural analysis plays a critical role in bridge engineering, ensuring bridges can withstand dynamic loads, such as traffic and natural forces. It involves evaluating stress distribution, material durability, and structural integrity to guarantee safety and longevity. Advanced techniques, like finite element analysis, are used to simulate real-world conditions, enabling engineers to design efficient and resilient structures. Bridge engineering relies on precise calculations to account for factors like weight distribution, environmental impacts, and long-term material behavior. By applying principles of structural analysis, engineers can create durable, cost-effective bridges that meet modern transportation demands while minimizing environmental impact.
Challenges in Structural Analysis
Structural analysis faces challenges like complex load conditions, material nonlinearity, and modeling inaccuracies, requiring advanced computational methods to ensure accurate predictions and reliable designs under various conditions.
Complex Load Conditions
Complex load conditions present significant challenges in structural analysis, as they involve multiple, varying forces acting on a structure simultaneously; These loads can be static, dynamic, or cyclic, and may include combinations of axial, torsional, and bending stresses. Analyzing such conditions requires advanced computational methods to accurately predict how structures behave under varied and extreme scenarios. Engineers must account for factors like load distribution, material behavior, and boundary conditions to ensure structural integrity. Modern tools, such as finite element analysis (FEA) software, enable detailed modeling of these complex interactions. Accurate prediction of stress distributions and deformations is critical to prevent failures and ensure safety. Overcoming these challenges is essential for designing resilient and efficient structures.
Material Nonlinearity
Material nonlinearity refers to the behavior of materials that deviates from linear elasticity, where stress is not proportional to strain. This occurs when materials exhibit plasticity, creep, or other inelastic responses under load. Nonlinear material behavior is particularly significant in extreme conditions, such as high stresses or large deformations, where materials may yield or fracture. Analyzing such behavior requires advanced computational models to capture the complex stress-strain relationships and failure mechanisms accurately.
Nonlinear material effects, such as strain hardening or softening, complicate structural analysis. Engineers must use specialized software to simulate these behaviors, ensuring designs account for potential material degradation or instability. Accurate modeling of material nonlinearity is critical for predicting structural performance under real-world conditions.
Future Trends in Structural Analysis
Future trends include advancements in computational methods, AI, and machine learning for simulations. Sustainable materials and energy-efficient designs are also expected to shape the field significantly.
Advancements in Computational Methods
Recent advancements in computational methods have revolutionized structural analysis, enabling more accurate and efficient simulations. Finite Element Method (FEM) and computational fluid dynamics (CFD) are now integrated with machine learning algorithms to predict material behavior and optimize designs. Cloud-based simulation tools allow engineers to analyze complex structures in real-time, reducing costs and improving collaboration. Additionally, AI-driven software automates iterative design processes, accelerating project timelines. These technologies also enable the analysis of large datasets, providing deeper insights into structural performance under various conditions. As computational power grows, so does the ability to model intricate systems, ensuring safer and more sustainable structures for the future.
Sustainable and Resilient Structures
Advancements in structural analysis are driving the development of sustainable and resilient structures. Modern techniques focus on optimizing material use, reducing environmental impact, and ensuring structures can withstand extreme conditions. Green building practices, such as energy-efficient designs and recycled materials, are increasingly incorporated. Resilience is enhanced through adaptive designs that account for climate change and natural disasters. Computational tools enable precise simulations to test sustainability and resilience. These innovations promote long-term durability, minimize resource consumption, and align with global sustainability goals, ensuring structures are both environmentally friendly and capable of enduring future challenges while maintaining functionality and safety.